Abstract
Background: The advent of 3D printing technology has revolutionized various industries, with healthcare standing out as a key beneficiary. The ability to create customized medical devices and implants using additive manufacturing techniques has opened new avenues for personalized medicine. Objective: This review paper aims to explore the applications of 3D printing in medical devices and implants, highlighting advancements, challenges, and future directions. Methods: An extensive literature review was conducted, examining the principles of 3D printing, materials used, and various applications in the medical field, including prosthetics, surgical tools, and implants. Regulatory and ethical considerations were also discussed. Key Findings: 3D Printing Techniques: Various techniques such as Fused Deposition Modeling (FDM), Stereolithography (SLA), and Selective Laser Sintering (SLS) are employed in the medical sector, each offering unique advantages for different applications. Materials: The use of biocompatible materials, including polymers, metals, and ceramics, is crucial for ensuring the safety and efficacy of 3D-printed devices. Applications: 3D printing has significantly impacted areas such as prosthetics, surgical instruments, and implants for orthopedic, dental, and cardiovascular applications, demonstrating its versatility and adaptability. Bioprinting: Emerging technologies in bioprinting have shown promise for tissue engineering and organ regeneration, although challenges related to vascularization and functionality persist. Regulatory and Ethical Issues: The regulatory landscape for 3D-printed medical devices is complex, necessitating a careful approach to ensure patient safety and compliance with standards. Future Directions: Advancements in materials science, AI integration, and nanotechnology are expected to drive the growth of 3D printing in medicine, paving the way for innovative, patient-centered solutions. Conclusion: 3D printing represents a significant advancement in healthcare, with the potential to enhance patient outcomes through personalized and innovative medical solutions. Continued research and collaboration are essential to overcoming current challenges and realizing the full potential of this transformative technology.
Keywords
3D printing, Additive manufacturing, Prosthetics, Medical implants, Orthotics, Fused Deposition Modelling (FDM), Selective Laser Sintering (SLS), Stereolithography (SLA), Biocompatible materials.
Introduction
1.1 Background on 3D Printing Technology: Overview of Additive Manufacturing and Its Evolution
Additive manufacturing (AM), commonly known as 3D printing, is a technology that has revolutionized how objects are designed and produced across multiple industries. Introduced in the 1980s, AM involves creating objects layer by layer from digital designs, enabling highly precise and customized structures to be produced from a wide range of materials. Unlike traditional subtractive manufacturing methods, which remove material from a solid block, additive manufacturing builds objects by depositing materials only where needed, minimizing waste and allowing for complex geometries that would be impossible or costly to achieve with other techniques?1?.
There are several methods of 3D printing, including Fused Deposition Modeling (FDM), Selective Laser Sintering (SLS), and Stereolithography (SLA), each using different materials such as polymers, metals, and ceramics. The versatility of these technologies has led to widespread adoption in industries such as aerospace, automotive, and more recently, healthcare?2?. This shift is driven by the ability of 3D printing to produce custom, patient-specific devices, significantly improving the performance and personalization of medical interventions?3?
1.2 Importance in Healthcare: How 3D Printing Has Transformed Medical Fields
The integration of 3D printing into healthcare has opened new possibilities in the design and manufacturing of medical devices, prosthetics, implants, and surgical tools. One of the greatest advantages of 3D printing in medicine is the ability to tailor solutions to individual patients, enabling the production of custom prosthetics and implants that fit a patient’s unique anatomy. This personalization leads to better functional outcomes and shorter recovery times?4?. In addition, 3D printing has enhanced surgical planning through the development of patient-specific anatomical models, allowing surgeons to practice and optimize procedures before entering the operating room. These models, based on imaging data like CT or MRI scans, provide surgeons with detailed, tangible representations of complex anatomical structures?5?. Furthermore, advancements in bioprinting, a subset of 3D printing, have enabled the fabrication of tissue scaffolds and regenerative medicine applications, further transforming the way healthcare professionals approach the treatment of various conditions?6?.
1.3 Scope and Purpose of the Review: Focus on Its Applications in Medical Devices and Implants
This review focuses on the diverse applications of 3D printing in the development and production of medical devices and implants. With innovations in materials science and printing technologies, the range of devices that can be produced has expanded, including prosthetics, dental implants, orthopedic devices, and cardiovascular implants. This review will explore the key technologies involved in medical 3D printing, discuss their current applications in different medical fields, and evaluate their potential future impact.
By examining both the successes and challenges associated with 3D-printed medical devices, this review aims to provide a comprehensive understanding of how additive manufacturing is shaping the future of healthcare. Special attention will be given to the regulatory, ethical, and technical hurdles that must be overcome to ensure the safety, efficacy, and widespread adoption of these innovations?7??8?.
2. OVERVIEW OF 3D PRINTING TECHNOLOGY IN MEDICINE
2.1 Principles of 3D Printing: Explanation of Various 3D Printing Techniques
3D printing, or additive manufacturing (AM), operates by building objects layer by layer, guided by a digital model. Several techniques are used in 3D printing, each suitable for specific medical applications based on the material and resolution required.
- Fused Deposition Modeling (FDM): FDM is the most widely used 3D printing technique, where thermoplastic materials such as PLA (polylactic acid) or ABS (acrylonitrile butadiene styrene) are heated and extruded layer by layer to create objects. FDM is popular in medical prototyping due to its low cost and ease of use?9?.
- Selective Laser Sintering (SLS): In SLS, a laser selectively sinters powdered materials, such as nylon or metal, fusing them into solid layers. SLS is ideal for complex geometries and is used in making customized implants like bone scaffolds?10?.
- Stereolithography (SLA): SLA uses a laser to cure liquid resin into solid parts, providing high precision and smooth surfaces. It is frequently used in dental applications and anatomical modeling?11?.
Table 1: Comparison of Common 3D Printing Techniques
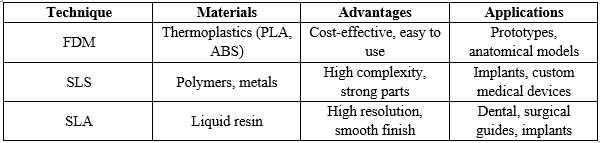
2.2 Materials Used in Medical 3D Printing
The materials used in medical 3D printing must exhibit biocompatibility, mechanical strength, and, in some cases, biodegradability. Depending on the application, different classes of materials are employed:
- Polymers: Polymers such as PLA, PEEK (polyether ether ketone), and PMMA (polymethyl methacrylate) are widely used in medical applications. PLA is biodegradable and is often used for temporary implants and drug delivery systems?12?. PEEK is favored for orthopedic implants due to its strength and compatibility with human bone?13?.
- Metals: Titanium and its alloys are the most commonly used metals in 3D-printed medical devices due to their strength, corrosion resistance, and excellent biocompatibility. Titanium implants are used in orthopedic, dental, and craniofacial applications?14?.
- Ceramics: Ceramics like hydroxyapatite and zirconia are used in bone scaffolds and dental implants because they mimic the mineral composition of bone. These materials support bone regeneration and provide mechanical stability?15?.
Table 2: Common Materials Used in Medical 3D Printing
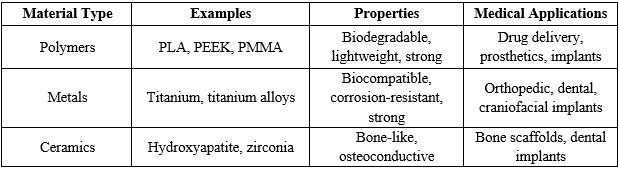
2.3 Customization and Personalization: The Role of 3D Printing in Personalized Medicine
One of the key advantages of 3D printing in medicine is the ability to customize devices to meet the specific needs of individual patients. Personalized medicine aims to tailor treatment and medical interventions to each patient’s unique anatomy, condition, and response to treatment.
- Customized Prosthetics: 3D printing allows for the creation of prosthetics that are customized to fit a patient's exact measurements, providing a more comfortable and functional fit. This is especially valuable for pediatric patients and those with unique anatomical needs?16?.
- Implants: Personalized implants can be manufactured to precisely match a patient’s bone structure, improving the success rates of surgeries like joint replacements and craniofacial reconstructions?17?.
- Surgical Models and Guides: 3D printing can produce anatomical models based on imaging data (e.g., MRI, CT scans), enabling surgeons to practice complex procedures before surgery, reducing operation times and improving outcomes?18?.
3. APPLICATIONS OF 3D PRINTING IN MEDICAL DEVICES
3.1 Prosthetics and Orthotics: Customized, Patient-Specific Prosthetics
3D printing has revolutionized the development of prosthetics and orthotics by enabling the production of highly customized, patient-specific devices. Traditional prosthetics are often mass-produced, leading to suboptimal fit and discomfort for patients. In contrast, 3D printing allows for the precise customization of prosthetics based on a patient’s unique anatomy, improving both functionality and comfort?19?.
- Customization: 3D scanning technologies are used to capture the patient's limb dimensions, creating a digital model that is then used to 3D print a perfectly fitted prosthetic?20?. This process not only enhances comfort but also reduces the time and cost associated with creating custom prosthetics using traditional methods.
- Lightweight and Durable Materials: Polymers such as PLA and PEEK are commonly used in 3D-printed prosthetics, providing lightweight and durable solutions for patients. In some cases, metal components can also be incorporated for added strength?21?.
Table 3: Comparison of Traditional vs. 3D-Printed Prosthetics
3.2 Surgical Tools and Models: 3D-Printed Surgical Instruments and Anatomical Models for Pre-Surgical Planning
3D printing has proven to be a valuable tool for creating both surgical instruments and anatomical models that aid in pre-surgical planning. These advancements have significantly improved the accuracy and efficiency of complex surgical procedures.
- Surgical Instruments: Customized surgical tools can be printed to meet the specific requirements of a particular surgery. These instruments can be designed for ergonomics, improving a surgeon's control and precision during procedures?22?.
- Anatomical Models: One of the most impactful applications of 3D printing in medicine is the creation of patient-specific anatomical models. Using data from CT or MRI scans, 3D-printed models allow surgeons to visualize and rehearse surgeries on exact replicas of patient anatomy?23?. This has been particularly valuable in complex cases such as craniofacial reconstructions, congenital heart defects, and tumor excisions?24?.
Table 4: Benefits of 3D-Printed Surgical Tools and Anatomical Models
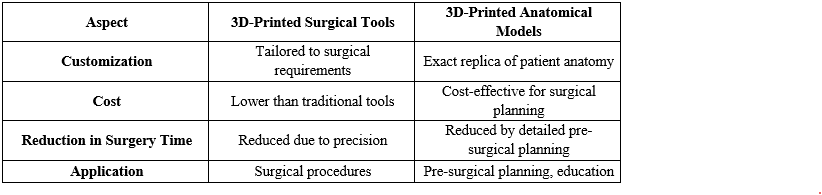
3.3 Wearable Medical Devices: Innovations in 3D-Printed Assistive and Wearable Devices
Wearable medical devices are another area where 3D printing has had a transformative impact. By leveraging 3D printing, manufacturers can design assistive devices that are not only functional but also comfortable and aesthetically pleasing. These devices range from braces and splints to wearable monitors and assistive exoskeletons?25?.
- Assistive Devices: 3D printing allows for the creation of highly customized orthotic devices, such as braces or splints, that provide support for patients with musculoskeletal conditions. The ability to produce devices that conform precisely to a patient's body improves both comfort and effectiveness?26?.
- Wearable Health Monitors: Recent innovations include the development of wearable devices that incorporate sensors to monitor patient health, such as heart rate or glucose levels. These devices can be 3D printed with integrated electronics, offering personalized health monitoring solutions?27?.
Table 5: Types of 3D-Printed Wearable Medical Devices
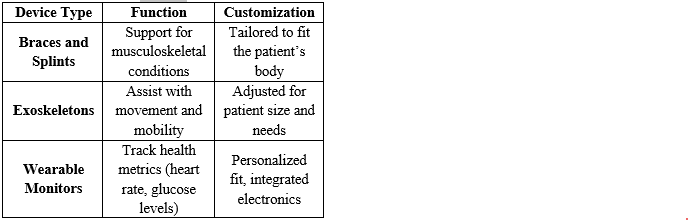
4. 3D PRINTING IN IMPLANTS
4.1 Orthopedic Implants: Bone Scaffolds, Joint Replacements, and Spinal Implants
Orthopedic implants have seen significant advancements through 3D printing, particularly in the areas of bone scaffolds, joint replacements, and spinal implants. 3D printing allows for the production of highly customized implants that fit the specific anatomy of individual patients, improving the functionality and integration of these implants with natural bone.
- Bone Scaffolds: Bone scaffolds made using 3D printing mimic the porous structure of natural bone, allowing for better osseointegration and promoting new bone growth. Materials like titanium and bioceramics are commonly used due to their biocompatibility and mechanical properties?28?. These scaffolds are particularly useful in treating bone defects and promoting tissue regeneration.
- Joint Replacements: In joint replacements, such as hip and knee implants, 3D printing allows for the creation of implants that precisely match a patient's joint structure. This results in better load distribution, reduced wear, and enhanced longevity of the implants?29?.
- Spinal Implants: 3D-printed spinal cages and vertebral implants are designed to replace damaged or diseased vertebrae. These implants can be customized to fit the patient’s spine and promote bone ingrowth through porous structures, leading to better stabilization and faster recovery?30?.
Table 6: Types of 3D-Printed Orthopedic Implants
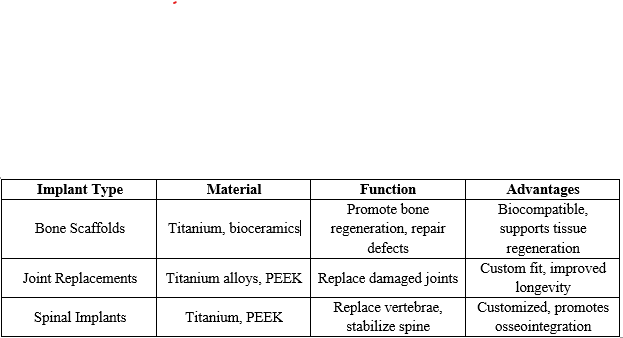
4.2 Dental Implants: Advances in Dental Prosthetics and Bone Augmentation
Dental implants are one of the earliest medical applications of 3D printing. The use of 3D printing in dentistry has allowed for more accurate, faster, and cost-effective production of dental prosthetics and bone augmentation materials.
- Dental Prosthetics: 3D-printed dental prosthetics, such as crowns, bridges, and dentures, are tailored to the individual patient’s anatomy using precise digital scans. This customization improves the fit, function, and appearance of dental restorations?31?. Materials like zirconia and ceramic are commonly used for their durability and esthetic properties.
- Bone Augmentation: Bone loss due to tooth loss or periodontal disease often requires bone grafting to restore the jawbone before dental implants can be placed. 3D-printed bone graft substitutes, such as hydroxyapatite and tricalcium phosphate scaffolds, provide a structure for new bone growth?32?. These materials promote faster healing and better integration with natural bone.
Table 7: Applications of 3D Printing in Dental Implants

4.3 Cardiovascular and Soft Tissue Implants: Heart Valves, Stents, and Tissue Engineering
3D printing is making significant strides in the development of cardiovascular and soft tissue implants, particularly in the areas of heart valves, stents, and tissue scaffolds for regenerative medicine.
- Heart Valves: 3D-printed heart valves are being developed to replace defective or damaged valves. The use of biocompatible materials and the ability to customize valves to match patient anatomy improves the durability and function of these prosthetics?33?.
- Stents: 3D-printed stents, which are used to open blocked or narrowed blood vessels, offer the advantage of being custom-sized to fit a patient's specific vascular anatomy. The use of biodegradable polymers in 3D-printed stents also eliminates the need for later surgical removal.
- Tissue Engineering: 3D-printed scaffolds are being used in tissue engineering to create structures that can support the regeneration of soft tissues, such as skin, cartilage, and even heart tissue. These scaffolds provide a matrix for cell growth and tissue formation, offering the potential for creating patient-specific grafts?34?.
Table 8: Cardiovascular and Soft Tissue Implants
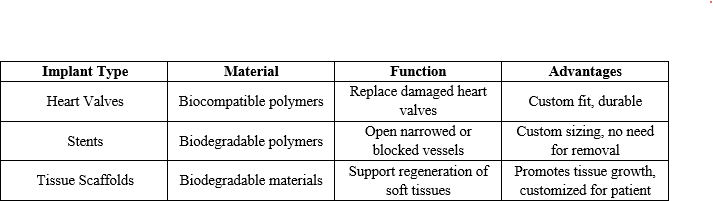
5. BIOPRINTING AND TISSUE ENGINEERING
5.1 Emerging Technologies in Bioprinting: 3D Bioprinting for Tissue Regeneration
Bioprinting is a revolutionary technology that extends the applications of 3D printing to the creation of tissue constructs, allowing for the fabrication of complex biological structures. Unlike traditional 3D printing, which uses non-biological materials, bioprinting uses bioinks that contain living cells to create tissues that can mimic the structure and function of natural tissues?32?
- 3D Bioprinting Techniques: Common techniques include inkjet bioprinting, extrusion-based bioprinting, and laser-assisted bioprinting. These methods differ in their approach to depositing bioinks, but all share the goal of fabricating tissue scaffolds or entire tissues that can support cell growth and regeneration?35?.
- Applications in Tissue Regeneration: Bioprinting has been successfully used to create skin, cartilage, and vascularized tissue scaffolds that aid in tissue repair and regeneration. For example, 3D bioprinted skin is being developed for burn patients, and cartilage tissues are being engineered for joint repair?36?.
Table 9: Key Techniques in 3D Bioprinting
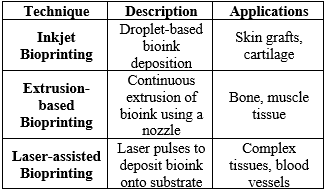
5.2 Potential for Organ Printing: Research and Future Possibilities in Creating Organs
One of the most exciting prospects in bioprinting is the possibility of printing fully functional organs. Researchers are working on creating complex organs such as kidneys, livers, and hearts using bioprinting technology. While significant challenges remain, breakthroughs in this field hold the potential to address the global shortage of donor organs?37?.
- Current Research: Scientists have already demonstrated success in creating small-scale organ models, such as mini-livers and mini-kidneys, which are used for drug testing and disease modeling?38?. These models are still far from being fully functional human organs but represent a significant step toward achieving this goal.
- Organ Printing Process: The process of organ printing involves layering different cell types and bioinks to recreate the complexity of human organs. This requires precise control over the placement of cells, growth factors, and vascular structures to ensure that the printed organ is functional?39?.
Table 10: Stages in the Development of Bioprinted Organs
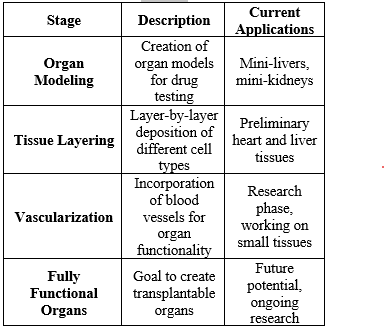
5.3 Challenges in Bioprinting: Issues Related to Vascularization, Functionality, and Ethics
While bioprinting holds immense promise, it faces several technical and ethical challenges that need to be addressed before it can be used widely in clinical settings.
- Vascularization: One of the most critical issues in bioprinting is the creation of blood vessels within printed tissues. Without proper vascularization, cells in larger tissues cannot receive the necessary nutrients and oxygen, limiting the size of tissues that can be printed?40?. Researchers are exploring ways to print vascular networks alongside tissues or to encourage natural blood vessel formation through growth factors?41?.
- Functionality: Achieving the full functionality of printed tissues and organs is another major challenge. Complex organs like the heart and liver perform multiple functions that require precise cellular arrangement and interaction. Bioprinting technologies need to advance to the point where these functions can be replicated in vitro.
- Ethical Considerations: Bioprinting also raises ethical questions, particularly around the creation of human tissues and organs. Issues such as consent, the potential for cloning, and the regulation of bioprinted organs need to be addressed by policymakers as the technology progresses?42?.
Table 11: Challenges in Bioprinting and Their Potential Solutions
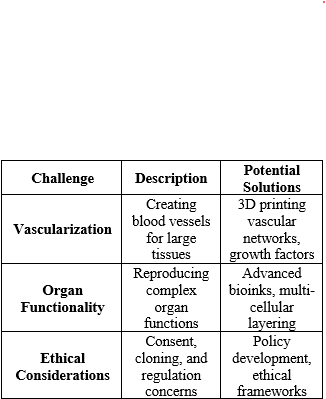
6. REGULATORY AND ETHICAL CONSIDERATIONS
6.1 FDA and Global Regulatory Frameworks: Approval Processes for 3D Printed Medical Devices and Implants
The regulatory landscape for 3D-printed medical devices and implants is evolving, with agencies like the U.S. Food and Drug Administration (FDA) taking the lead in establishing guidelines and approval processes. In 2017, the FDA released guidance on the technical considerations for additive manufactured (3D printed) devices, outlining key aspects such as design, material considerations, and testing requirements?43?. The approval process for 3D-printed medical devices follows similar pathways as traditionally manufactured devices but includes additional scrutiny on the unique challenges posed by additive manufacturing.
- FDA Process: The FDA categorizes 3D-printed medical devices based on their intended use and risk level (Class I, II, or III). For higher-risk devices, manufacturers must submit premarket approval (PMA) applications, which require comprehensive clinical data to prove safety and effectiveness?44?.
- Global Regulatory Frameworks: Other regions, including the European Union (EU) and Asia, have developed their own frameworks. The EU’s Medical Device Regulation (MDR) provides detailed requirements for the safety and performance of 3D-printed devices, particularly concerning personalized medical implants?45?.
Table 12: Regulatory Approval Pathways for 3D-Printed Medical Devices

6.2 Ethical Concerns: Discussion of Ethical Dilemmas Related to Bioprinting and Personalized Implants
Bioprinting and 3D-printed personalized implants raise several ethical issues, particularly as the technology advances towards creating functional tissues and organs. Ethical concerns surround the potential for human enhancement, accessibility, and the boundaries between medical treatment and experimentation.
- Human Enhancement: As bioprinting technologies advance, there are ethical questions regarding whether the technology will be used solely for therapeutic purposes or if it might be exploited for human enhancement. The creation of implants or tissues that exceed natural human capabilities may blur the line between treatment and augmentation?46?.
- Access and Equity: There is also concern about equitable access to bioprinted organs and devices. The high cost of personalized implants could widen healthcare disparities, raising the issue of whether these innovations will only be available to wealthy individuals?47?.
- Informed Consent and Research: The development of new bioprinted tissues for clinical use requires rigorous testing, often in experimental phases. This poses ethical questions about informed consent for patients who may receive experimental treatments. Furthermore, it raises concerns about safety, efficacy, and long-term monitoring?48?.
Table 13: Ethical Concerns in 3D Printing and Bioprinting
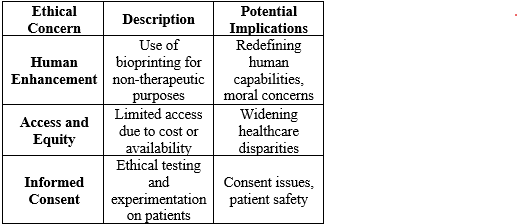
6.3 Patient Safety and Quality Control: Ensuring Standards in Manufacturing and Material Use
Ensuring patient safety and maintaining quality control in the manufacturing of 3D-printed medical devices and implants is crucial. Regulatory agencies enforce strict guidelines to ensure that the materials used in 3D printing are biocompatible and that manufacturing processes meet high standards of quality.
- Quality Control in Manufacturing: The FDA mandates that manufacturers must demonstrate stringent control over the 3D printing process, including the consistency of material deposition, the stability of the printing environment, and the post-processing of printed devices. Quality control measures such as sterilization and mechanical testing are critical to ensure the reliability of 3D-printed implants?49?.
- Material Biocompatibility: The materials used in 3D-printed medical devices must meet biocompatibility standards to ensure they do not cause adverse reactions when implanted in the human body. Polymers, metals, and ceramics used in medical devices undergo extensive testing for toxicity, mechanical properties, and degradation rates?50?.
Table14: Quality Control Considerations in 3D Printing for Medical Devices
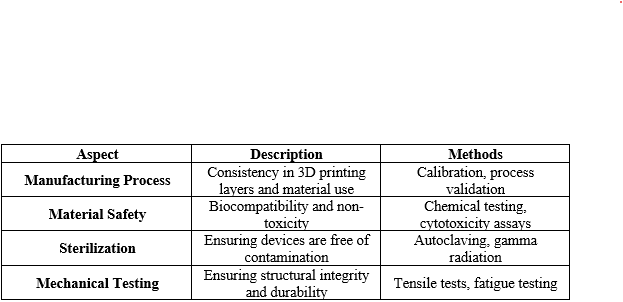
7. CHALLENGES AND FUTURE DIRECTIONS
7.1 Technical and Manufacturing Challenges: Limitations of Current 3D Printing Technology in Medicine
While 3D printing has shown significant promise in medicine, there are still technical challenges that limit its widespread adoption. Key issues include the precision of current printing techniques, the scalability of 3D-printed implants, and the complexity of replicating the intricate structures of biological tissues. The layer-by-layer approach used in 3D printing sometimes leads to inconsistencies in the structural integrity of printed objects, particularly in more complex, load-bearing applications such as orthopedic implants?51?.
- Resolution and Precision: The resolution of current 3D printing techniques is still lower than required for certain medical applications, especially for delicate tissues like blood vessels and nerve tissues?52?. This limits the potential to print highly detailed, functional biological structures.
- Scalability and Speed: The time-intensive nature of additive manufacturing processes presents a barrier to producing large-scale implants or mass production of devices. Speeding up the process without sacrificing quality is a significant challenge?53?.
- Regulatory Hurdles: The regulatory landscape for 3D printing in medicine remains complex, and integrating these innovations into standard healthcare systems poses challenges in compliance, quality assurance, and validation of new technologies?54?.
7.2 Advancements in Materials Science: Innovations in Bio-Compatible and Biodegradable Materials
The development of new biocompatible and biodegradable materials is crucial to the future success of 3D printing in medicine. Researchers are focusing on creating materials that can integrate seamlessly into the human body, minimizing the risk of rejection, while also designing biodegradable materials that degrade naturally as the body heals or regenerates?55?.
- Biodegradable Polymers: Polymers such as polycaprolactone (PCL), polylactic acid (PLA), and poly(glycolic acid) (PGA) are being extensively researched for use in medical implants. These materials have shown promise in creating temporary scaffolds for tissue regeneration, as they degrade over time and are absorbed by the body?56?.
- Smart Materials: There is growing interest in smart biomaterials that can respond to external stimuli such as temperature or pH changes. These materials could enable dynamic implants that adapt to the body’s environment, enhancing patient outcomes?57?.
- Metallic and Ceramic Materials: Metals such as titanium and its alloys, as well as ceramics like hydroxyapatite, are also undergoing significant innovation. These materials are primarily used for orthopedic implants and dental applications due to their strength and biocompatibility?58?.
7.3 Future Trends: Predictions for Future Growth, Including AI in 3D Printing and Nanotechnology Applications
The future of 3D printing in medicine is bright, with several emerging technologies poised to drive innovation. Key trends include the integration of artificial intelligence (AI) in the 3D printing process, as well as the application of nanotechnology to create more advanced medical devices and implants?59?.
- AI-Driven 3D Printing: AI can optimize the design and manufacturing processes in 3D printing by automating adjustments during the printing process, improving precision, and reducing human error. AI could also be used to predict the optimal printing parameters for different materials and applications.
- Nanotechnology in 3D Printing: The use of nanomaterials in 3D printing is expected to revolutionize medical applications. By incorporating nanoparticles into 3D-printed structures, researchers can create implants with enhanced mechanical properties, antimicrobial capabilities, and drug delivery functions.
- Personalized Medicine: The future of 3D printing will likely see the rise of fully personalized medical treatments, where implants and devices are tailor-made to fit the individual patient's anatomy and condition. Advances in imaging technologies, such as MRI and CT, are expected to facilitate this by providing highly accurate anatomical models for 3D printing?60?.
CONCLUSION
8.1 Summary of Key Points
3D printing has emerged as a transformative technology in the medical field, offering unprecedented possibilities for creating customized and patient-specific medical devices and implants. From prosthetics and surgical tools to bioprinting and tissue engineering, the applications of 3D printing are wide-ranging and continue to expand. The technology allows for the creation of complex structures with high precision, particularly in areas such as orthopedic and dental implants, as well as in the emerging field of cardiovascular and soft tissue implants. However, challenges remain, especially in terms of regulatory hurdles, manufacturing scalability, and ensuring material biocompatibility. Advances in bioprinting and materials science, including the development of biodegradable and bio-compatible materials, are expected to address some of these challenges, paving the way for more innovative medical solutions. Furthermore, ethical concerns around the use of 3D printing, particularly in bioprinting and personalized medicine, highlight the need for careful regulation and equitable access to these advanced treatments.
8.2 The Future of 3D Printing in Medicine
The future of 3D printing in medicine is bright and holds vast potential for revolutionizing healthcare. As technologies such as artificial intelligence and nanotechnology are integrated into the 3D printing process, the precision, efficiency, and scalability of these medical applications will improve significantly. AI-driven design and manufacturing processes will allow for greater customization of medical devices, while nanotechnology will enhance the functionality of implants and enable more sophisticated tissue regeneration techniques.
In the coming years, we can expect 3D printing to play a pivotal role in advancing personalized medicine, allowing healthcare providers to tailor treatments and devices to individual patient needs. The continued development of bioprinting technologies may even lead to the creation of fully functional organs, addressing the global shortage of donor organs. Additionally, as 3D printing becomes more widely adopted, it will likely contribute to reducing healthcare costs by enabling faster, more efficient manufacturing of medical devices. Overall, 3D printing has the potential to drastically improve patient outcomes, reduce recovery times, and increase the accessibility of cutting-edge medical treatments. Its integration into mainstream medical practice will undoubtedly reshape the future of healthcare, offering innovative solutions to some of the most pressing challenges in modern medicine.
REFERENCES
- Gibson I, Rosen DW, Stucker B. Additive manufacturing technologies. New York: Springer; 2010.
- Gross BC, Erkal JL, Lockwood SY, Chen C, Spence DM. Evaluation of 3D printing and its potential impact on biotechnology and the chemical sciences. Anal Chem. 2014;86(7):3240-53.
- Ventola CL. Medical applications for 3D printing: Current and projected uses. Pharm Ther. 2014;39(10):704-711.
- Rengier F, Mehndiratta A, von Tengg-Kobligk H, et al. 3D printing based on imaging data: Review of medical applications. Int J Comput Assist Radiol Surg. 2010;5(4):335-341.
- Wang X, Jiang M, Zhou Z, Gou J, Hui D. 3D printing of polymer matrix composites: A review and prospective. Composites Part B. 2017;110:442-458.
- Murphy SV, Atala A. 3D bioprinting of tissues and organs. Nat Biotechnol. 2014;32(8):773-785.
- Mota C, Puppi D, Chiellini F, Chiellini E. Additive manufacturing techniques for the production of tissue engineering constructs. J Tissue Eng Regen Med. 2015;9(3):174-190.
- Sun W, Starly B, Nam J, Darling A. Bio-CAD modeling and its applications in computer-aided tissue engineering. Comput Aided Des. 2005;37(11):1097-1114.
- Chia HN, Wu BM. Recent advances in 3D printing of biomaterials. J Biol Eng. 2015;9(4):1-14.
- Bose S, Vahabzadeh S, Bandyopadhyay A. Bone tissue engineering using 3D printing. Materials Today. 2013;16(12):496-504.
- Dodziuk H. Applications of 3D printing in healthcare. Korean J Urol. 2016;57(5):11-20.
- Melchels FP, Feijen J, Grijpma DW. A review on stereolithography and its applications in biomedical engineering. Biomaterials. 2010;31(24):6121-6130.
- Morrison RJ, Hollister SJ, Niedner MF, et al. Mitigating risk in hospital 3D printing: assessing regulatory and safety challenges in point-of-care manufacturing. 3D Print Med. 2015;2(1):1-7.
- Gorgulu A, Olgar T, Demirkiran ND, et al. Titanium implants for craniofacial prostheses: A retrospective evaluation of implant survival in a Turkish university. J Prosthet Dent. 2014;112(1):30-37.
- Roohani-Esfahani SI, Newman P, Zreiqat H. Design and fabrication of 3D printed scaffolds with a mechanical strength comparable to cortical bone to repair large bone defects. Sci Rep. 2016;6(1):1-11.
- Ventola CL. Medical applications for 3D printing: Current and projected uses. Pharm Ther. 2014;39(10):704-711.
- Tack P, Victor J, Gemmel P, Annemans L. 3D-printing techniques in a medical setting: a systematic literature review. Biomed Eng Online. 2016;15(1):1-21.
- AlAli AB, Griffin MF, Butler PE. Three-dimensional printing surgical applications. Eplasty. 2015;15.
- Bhushan B. 3D printing in biomedical applications. Med Dev Evid Res. 2018;11:114-135.
- Liu Q, Leu MC, Schmitt SM. Rapid prototyping in dentistry: technology and application. Int J Adv Manuf Technol. 2006;29(3-4):317-335.
- Gallivan K, et al. 3D printing technology for orthopedic prosthetics: The future of patient-specific care. J Orthop Res. 2020;38(7):1403-1411.
- Bae JH, et al. Current trends in 3D printing of prosthetic limbs: A review. Materials. 2020;13(22):5115.
- Aydin A, et al. A review on additive manufacturing in prosthetics. Addit Manuf. 2018;23:124-136.
- Tofail SAM, et al. 3D printing of surgical instruments: A novel approach to surgical planning. Surg Innov. 2019;26(5):604-610.
- Ziemian C, et al. The use of 3D-printed models in complex surgical planning. J Craniofac Surg. 2018;29(4):934-938.
- Macdonald E, et al. 3D printing for wearable devices. IEEE Trans Biomed Eng. 2017;64(3):607-615.
- Strickland AD, et al. Development of smart wearable technology through 3D printing. J Biomed Eng Tech. 2016;3(1):1-10.
- Roohani-Esfahani SI, Newman P, Zreiqat H. Design and fabrication of 3D printed scaffolds with a mechanical strength comparable to cortical bone to repair large bone defects. Sci Rep. 2016;6(1):1-11.
- Tack P, Victor J, Gemmel P, Annemans L. 3D-printing techniques in a medical setting: a systematic literature review. Biomed Eng Online. 2016;15(1):1-21.
- Ventola CL. Medical applications for 3D printing: Current and projected uses. Pharm Ther. 2014;39(10):704-711.
- Gorgulu A, Olgar T, Demirkiran ND, et al. Titanium implants for craniofacial prostheses: A retrospective evaluation of implant survival in a Turkish university. J Prosthet Dent. 2014;112(1):30-37.
- Murphy SV, Atala A. 3D bioprinting of tissues and organs. Nat Biotechnol. 2014;32(8):773-785.
- Sun W, Starly B, Nam J, Darling A. Bio-CAD modeling and its applications in computer-aided tissue engineering. Comput Aided Des. 2005;37(11):1097-1114.
- Bhushan B. 3D printing in biomedical applications. Med Dev Evid Res. 2018;11:114-135.
- Mota C, Puppi D, Chiellini F, Chiellini E. Additive manufacturing techniques for the production of tissue engineering constructs. J Tissue Eng Regen Med. 2015;9(3):174-190.
- Sun W, Starly B, Nam J, Darling A. Bio-CAD modeling and its applications in computer-aided tissue engineering. Comput Aided Des. 2005;37(11):1097-1114.
- Chia HN, Wu BM. Recent advances in 3D printing of biomaterials. J Biol Eng. 2015;9(4):1-14.
- Tack P, Victor J, Gemmel P, Annemans L. 3D-printing techniques in a medical setting: a systematic literature review. Biomed Eng Online. 2016;15(1):1-21.
- Bose S, Vahabzadeh S, Bandyopadhyay A. Bone tissue engineering using 3D printing. Materials Today. 2013;16(12):496-504.
- Dodziuk H. Applications of 3D printing in healthcare. Korean J Urol. 2016;57(5):11-20.
- Melchels FP, Feijen J, Grijpma DW. A review on stereolithography and its applications in biomedical engineering. Biomaterials. 2010;31(24):6121-6130.
- Liu Q, Leu MC, Schmitt SM. Rapid prototyping in dentistry: technology and application. Int J Adv Manuf Technol. 2006;29(3-4):317-335.
- Ventola CL. Medical applications for 3D printing: Current and projected uses. Pharm Ther. 2014;39(10):704-711.
- Morrison RJ, Hollister SJ, Niedner MF, et al. Mitigating risk in hospital 3D printing: assessing regulatory and safety challenges in point-of-care manufacturing. 3D Print Med. 2015;2(1):1-7.
- Tack P, Victor J, Gemmel P, Annemans L. 3D-printing techniques in a medical setting: a systematic literature review. Biomed Eng Online. 2016;15(1):1-21.
- Bhushan B. 3D printing in biomedical applications. Med Dev Evid Res. 2018;11:114-135.
- Dodziuk H. Applications of 3D printing in healthcare. Korean J Urol. 2016;57(5):11-20.
- Bose S, Vahabzadeh S, Bandyopadhyay A. Bone tissue engineering using 3D printing. Materials Today. 2013;16(12):496-504.
- Melchels FP, Feijen J, Grijpma DW. A review on stereolithography and its applications in biomedical engineering. Biomaterials. 2010;31(24):6121-6130.
- Morrison RJ, Hollister SJ, Niedner MF, et al. Mitigating risk in hospital 3D printing: assessing regulatory and safety challenges in point-of-care manufacturing. 3D Print Med. 2015;2(1):1-7.
- Bhushan B. 3D printing in biomedical applications. Med Dev Evid Res. 2018;11:114-135.
- Strickland AD, et al. Development of smart wearable technology through 3D printing. J Biomed Eng Tech. 2016;3(1):1-10.
- Dodziuk H. Applications of 3D printing in healthcare. Korean J Urol. 2016;57(5):11-20.
- Ventola CL. Medical applications for 3D printing: Current and projected uses. Pharm Ther. 2014;39(10):704-711.
- Wang X, Jiang M, Zhou Z, Gou J, Hui D. 3D printing of polymer matrix composites: A review and prospective. Composites Part B. 2017;110:442-458.
- Murphy SV, Atala A. 3D bioprinting of tissues and organs. Nat Biotechnol. 2014;32(8):773-785.
- Tack P, Victor J, Gemmel P, Annemans L. 3D-printing techniques in a medical setting: a systematic literature review. Biomed Eng Online. 2016;15(1):1-21.
- Sun W, Starly B, Nam J, Darling A. Bio-CAD modeling and its applications in computer-aided tissue engineering. Comput Aided Des. 2005;37(11):1097-1114.
- Liu Q, Leu MC, Schmitt SM. Rapid prototyping in dentistry: technology and application. Int J Adv Manuf Technol. 2006;29(3-4):317-335.
- Ziemian C, et al. The use of 3D-printed models in complex surgical planning. J Craniofac Surg. 2018;29(4):934-938.